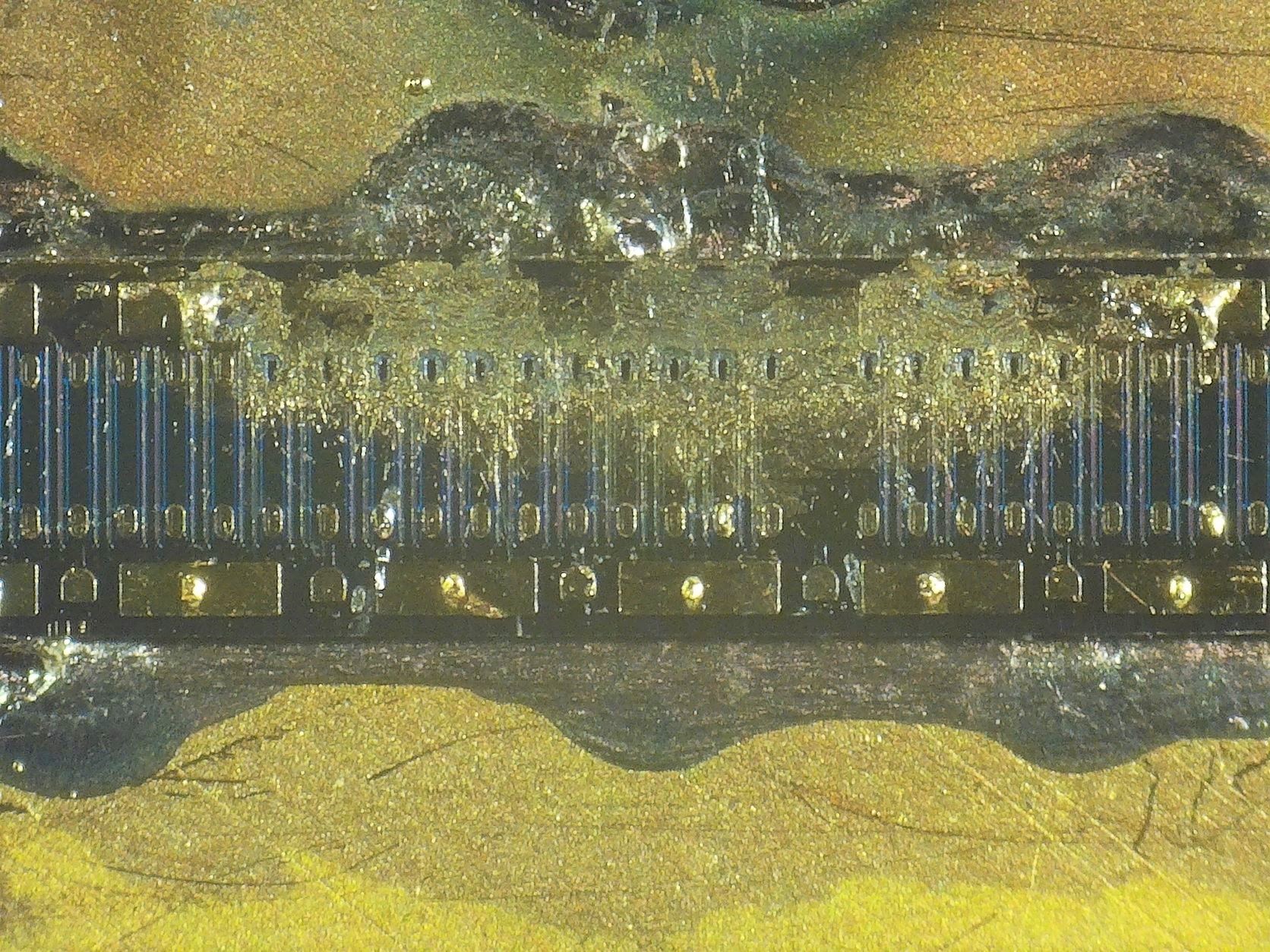
10W GaN burnt die
Peering underneath the hermetically-sealed ceramic lid from a discrete GaN HEMT transistor we can appreciate the device in all its glory. Inside sits a small piece of semiconductor, the bare die, which is mounted in the ceramic and metal packaging. The bare die is interconnected to the package using bond wires. The left bond wires connect to the gate (or input) while the right bound wires connect to the drain (or output) of the transistor. The bond wires connected to the metal plate beneath the bare die are the source. This bare die consists simple of a multi-finger HEMT transistor structure and has no on-chip matching.
We push our devices to the limit but in that process of discovery, transistors burn out… and that blows. That’s why it’s very important to have various protections in place to prevent that from happening. On this burnt specimen, we can do a little ad-hoc “failure analysis”. Fortunately, the gate bond wires are still intact but the drain side didn’t fare as well and those little gold threads seemed to have vaporized. Cleaning up the die a little bit gives us a better view of what happened on the visual level.
Be careful not to overdrive or over bias your GaN HEMT devices. They are sensitive creatures and require the utmost care.
After the smoke clears on this blown GaN HEMT, we can gain a better understanding of the situation by looking underneath the ceramic lid. The once smoke-filled package now leaves behind an interior that coated in soot. After cleaning it up a bit, we see that the lid took a direct hit from the transistor blow up but it is very well intact, though stained from the failure. The pattern of damage is consistent with the drain side of the transistor and bond wire area and shows some solder or vaporized bond wire splatter.
Transistor failures need to be investigated, otherwise they are destined to repeat. Echoic Engineering is highly experienced in the handling, characterization and analysis of RF / microwave GaN devices.
250W GaN burnt die pulsed
We wanted to find out what happens when you drive a pulsed power GaN device with CW and this little one rated at 250W pulsed certainly didn’t disappoint. In a matter of seconds, $300 turned into vapor, sort of! Looking under its ceramic hood and we can see the carnage left behind! Bond wires vaporized, extra heavy charcoal and even solder managed to seep into the packaging.
Cleaning it up we can see that this device consists of a bare die GaN HEMT transistor, bare die capacitor and bond wire interconnect. This little web of components forms a series inductor, shunt cap, series inductor distributed input matching network! Perfect for the wide periphery device consisting of 8 unit element transistors.
How do you know when your transistor is dead? Well, if you’re lucky, you’ll be able to catch the failure using real-time measurements. Burned transistors are sad but on the bright side, it does resemble art!
Performing a Pin-Pout sweep on this L-Band amplifier, we can see that it pumps out considerable CW power! Not bad for a pulsed-only GaN HEMT! The device easily generates 100W+ (50dBm+) but peaks out at 141W (51.5 dBm) before experiencing a catastrophic failure (0W). That’s how you know it’s a goner!
Taking a closer look at our GaN HEMT we can see some very interesting features! The region of most despair shows burnout around the vias on the output side. Can anyone guess why these failures take place near the center of the die? Yes, it’s related to the thermal profile along the chip!
It’s very important to detect and analyze transistor failures based on measurement. This will save development time and energy without having to open them up.
200W GaN burnt die CW
We often think of ICs and as black packages with exposed electrical contacts. In reality, the IC “black box” that you bought from Digikey actually contains many different interconnected components. These might consist of bare die (what the EE would call an IC), SMTs, PCBs and more. Furthermore, the mounting, interconnect and encapsulation of these parts is a topic of it’s own.
Inspection of this packaged 200W GaN device reveals four main pieces: two bare GaN HEMT die and two die for impedance matching. These parts are mounted using conductive die-attach with good thermal properties and are connected using bondwires (only landings shown). Technically this can be called a multi-chip hybrid or multi-chip-module (MCM). The entire assembly has been encapsulated in a plastic overmold to reduce cost at the expense of increased parasitic loss.
RFIC and MMIC development is a complex process and every piece of the device needs to be thoroughly analyzed for electrical and thermal properties along with size and cost. Echoic is highly experienced in the analysis and design of RF / microwave GaN devices.
After an extended time pushing out a whopping 200W CW RF signal, this GaN HEMT transistor decided to call it quits! As the brother of the transistor we last posted (not the exact same device) it saw some real action but soon became a burnt transistor 🙁
We’re showing the bottom mounting plate with the toasted bare die as well as an underside view of the top encapsulation mirrored over. One side has burned out before the other in this “gemini” package. Unlike the ceramic packaged breed, there is no soot and the bondwires were not vaporized. Rather, they have been fossilized into the encapsulation and are poking “into the page” (illustrated). What’s interesting is how much is “captured” inside the mold including an imprint of the GaN die themselves!
Developing high power GaN HEMT power amplifiers and pushing technology to the limits always comes with risks.